Brownian Motion of Ellipsoidal Particles
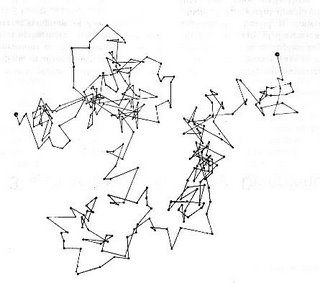
On average, particles undergoing Brownian motion do not move very far. For example, in one second, the largest number of particles will stay very close, say within one micron, of their starting point; a smaller number will move between one micron and two microns; a still smaller number will move between two microns and three microns, and so on. A plot of the number of particles traveling specific distances yields the famous bell-shaped or Gaussian curve from statistics.

On the other hand, the rotation of non-spherical particles may affect their translation. Since most Brownian particles are not spherical, they may experience cross-talk between translation and rotation. This idea of such a coupling was first published by French physicist Francis Perrin in the 1930s but were apparently "forgotten" by the science community. Perrin's father, Jean Perrin won the Nobel Prize in 1926 for the first experimental observations confirming Einstein's theories about Brownian motion.
In a recent research paper published in Science, a research team led by Arjun G. Yodh of University of Pennsylvania confirmed the theory's curious description of how an ellipsoid's random motions are different from those of spherical particles. They reported definitive measurement of the Brownian motion of an isolated ellipsoidal particle. UPenn researchers employed state-of-art digital imaging technology and computer image analysis for their experiments. Using a charge-couple device (CCD) camera, they recorded the orientations and positions of a single, micrometer-sized plastic ellipsoid particle suspended in water at a sequence of times. Their study of the motion produced a curve that is not Gaussian -- thus directly confirming ideas about rotational-translational coupling.
Reference: "Brownian Motion of an Ellipsoid" by Y. Han, A. M. Alsayed, M. Nobili, J. Zhang, T. C. Lubensky, and A. G. Yodh, Science 27 October 2006: pp626-630. Abstract.
Labels: Complex System, Einstein